Lifestyle Programs Tailored to Your Unique Genetic Profile
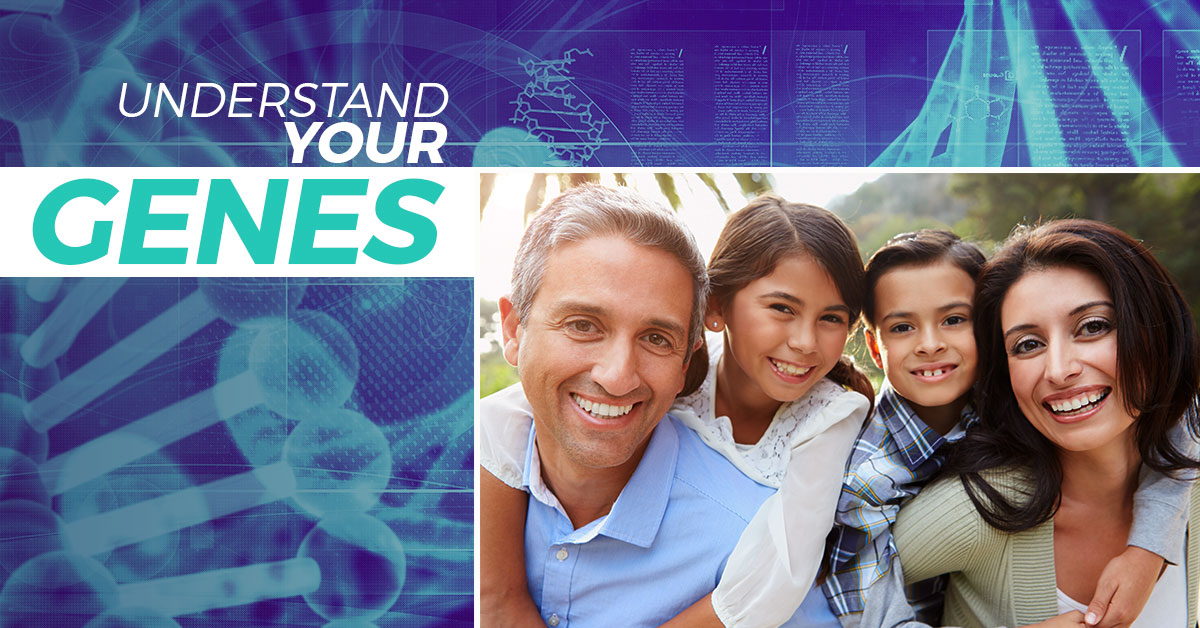
Understand Your Genes
ACE – Endurance/Power
What is the ACE gene?
More than 20 years ago, geneticists discovered a gene function that showed a substantial impact on human athletic performance. The angiotensin I-converting enzyme (commonly referred to as the ACE gene), first highlighted an impact on athletic performance. ACE is well associated with the renin-angiotensin system (RAS), which is the hormone system that regulates salt and fluid balance and blood pressure in the body. RAS functions within local cells and tissue where it cuts proteins. The ACE gene cuts angiotensin I and converts it to angiotensin II.
Most RAS functions and components have been identified as functional polymorphic variants. The most understood variant within the RAS is the ACE gene. When ACE inserts itself, there is an insertion/deletion (I/D) that research has shown to be connected to an improved performance and duration of exercise across a number of populations.
The I allele has consistently shown to play a role in improving athletic performance that relies heavily on endurance, such as in marathon runners. The D allele, however, has shown to be associated with improving both power and strength performance, such as in swimmers.
The ACE gene may be brought about by variants in loci or relation to genetic polymorphism like the growth hormones and the angiotensin receptor. However, these growth hormones have not shown to be significantly associated with performance in studies that focused on both military personnel and endurance athletes.
The ACE genotype also influences bradykinin levels, which are inflammatory mediators. This mediator is associated with increased endurance performances, as bradykinin levels are a common gene variant in bradykinin 2 receptors. These high levels of bradykinin haplotype has proven to be associated with increased performance in Olympics. It has also shown that they are associated with improved metabolic efficiency in triathletes.
Furthermore, in both healthy and diseased states, the ACE genotype changes when stimulated, specifically with the left ventricular mass. The I allele has low response to cardiac growth, while the D allele shows an exaggerated response to training. The connection of the I allele to endurance performance leads researchers to believe that other regulatory mechanisms exist. On the same page, the D allele responds to training with greater strength gains. Altogether, this means metabolic efficiency may be influenced by the ACE genotype. Better metabolism means improved athletic performance.
In short, the ACE genotype has shown to be associated with improved overall physical performance. However, the ACE genotype and closely related polymorphism are associated at the single organ level.
AGTR2 – Endurance/Power
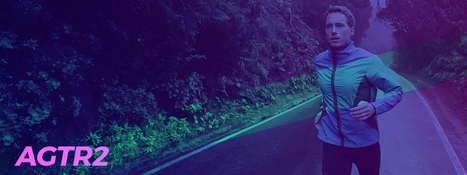
Muscle fiber composition is an integral part of not only athletic performance but overall movement and the ability to perform these movements with varying degrees of fiber composition in individuals. While there is quite a range of variation, endurance athletes have to have significantly high proportions of type 1 muscle fibers. Type 1 muscle fibers are associated with slow-twitch, fatigue-resistant fibers, making this muscle fiber type particularly beneficial for athletes.
Weightlifters and sprinters have different muscle fibers than endurance athletes. The latter has Type 1, as previously mentioned, while the sprinters and weightlifters have type IIA muscle fibers. These fibers signal responses for fast twitch and resistance to fatigue. Additionally, these athletes also have type IIX that is also responsible for fast twitch, but focuses on glycolytic response instead of fatigue resistance.
There’s an indication that one’s genotype and environment both determine the proportion of type I fibers in muscles, ranging from 40-50 percent. Individual, sequential variations in the DNA can vary because of a genotype interaction with the environment. Genetic variance, explained here, combines the effects of how genes interact alone, how genes interact with their environment, and how genes interact with each other.
Currently, understood determinants of muscle fiber types in humans involve the different types of training intensity, innervation, spacelift and unloading, thyroid levels, and disease. This has allowed researchers to better understand and identify signaling pathways that control these muscle fiber types.
Evidence suggests that DNA polymorphisms that influence gene expression within these signaling pathways predisposes cells’ muscle precursors within an individual to be predominantly fast or slow. This suggests that gene variations may be considered molecular determinants that maintain slow or fast myosin heavy chain (MyHC) expression of the adult skeletal muscle.
While studies on AGTR2 variation are few, research is showing promising evidence that the gene is associated with muscle fiber composition, affecting athletic and aerobic performance. Research shows that the C allele of AGTR2 appears to be associated with aerobic performance and athletic endurance through an increase in slow-twitch muscle fibers. Additionally, the A allele of AGTR2 is connected to fast-twitch fibers and athletic performance that heavily demands power, such as weightlifting and sprinting.
In summation, data suggest that the AGTR2 C allele is likely advantageous for athletes who participate in endurance athletics for both men and women, while the AGTR2 A allele is in relation to women and power sports.
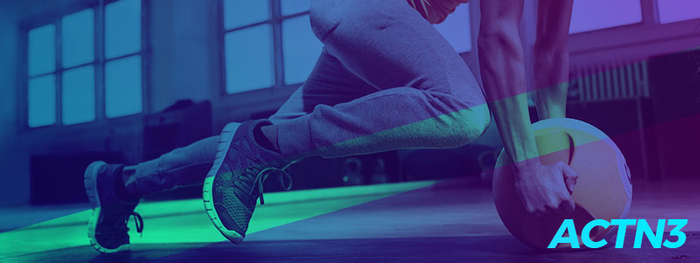
ACTN3 – Fast Twitch/Slow Twitch
Studies on α-actinin-3, commonly known as ACTN3, strongly suggest that, when a common polymorphism (R577X) is found in the gene, this results in a total deficiency of α-actinin-3 in roughly 16 percent of humans across the globe. However, α-actinin-3’s protein presence shows association with improved sprinting and power performance in athletes across the general population.
Studies have also shown that the null genotype XX, when acted on by recent positive selection, is most likely due to its role in regulating muscle metabolism. A deficiency of α-actinin-3, however, reduces glycogen phosphorylase activity, resulting in the energy utilization of more oxidative pathways.
Current data suggest α-actinin-3 is responsible for significant muscle metabolism regulation. A deficiency in fast-fiber skeletal muscle protein is somewhat common. This is owed to a polymorphic-null allele in the ACTN3 gene. Supported by numerous studies, this gene has shown to be detrimental to performance in both sprinting and power sports within the general population. A deficiency in this gene signals a characteristic shift of fast glycolytic muscle fibers towards slower muscle fibers with oxidative metabolism.
The ACTN3 has shown to be strongly associated with exceptional athletic performance. When compared to control groups, there is a highly significant reduction in the frequency of α-actinin-3-deficient persons who participate in sprinting and power sport.
PPARGC1A – How You Create Mitochondria
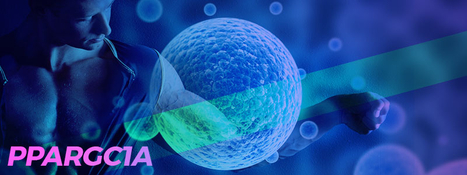
The protein PGC-1α (Peroxisome proliferator-activated receptor gamma coactivator 1-alpha) in encoded by the PPARGC1A gene and is the primary regulator of mitochondrial biogenesis.
PGC-1α is a transcriptional coactivator, meaning that it is responsible for regulating the genes that are involved with energy metabolism. It interacts with the nuclear receptor PPAR-γ, allowing for the interaction between the protein and numerous transcription factors. The protein contributes a direct link between outside physiological stimuli and regulating mitochondrial biogenesis.
Specifically, PGC-1α can also adjust individual mitochondria’s composition and functions, meaning that there is cellular remodeling by way of mitochondrial biogenesis and remodelling of organelles by way of alteration in elemental mitochondrial properties.
Increased PGC-1α has shown to be associated with an increase in reactive oxygen species (ROS) that largely come about by mitochondria. Recent studies, however, suggest that PGC-1α is an important regulator of ROS removal through increased ROS-detoxifying enzymes’ expression. Ultimately, this suggests that PGC1-α may control initiation of both mitochondrial metabolism and removal of its ROS waste, thus elevating oxidative metabolism and minimize ROS’ impact on the physiology of cells.
Ultimately, PGC-1α is largely responsible for universal global aerobic metabolism by presiding over both organelle biogenesis and remodelling. This is particularly significant in endurance athletes, as it is induced by endurance exercise. Contemporary research highlights that PGC-1α determine lactate metabolism, meaning that lactic acid levels in endurance athletes remain lower, thus resulting in a more efficient energy source for endurance athletes.
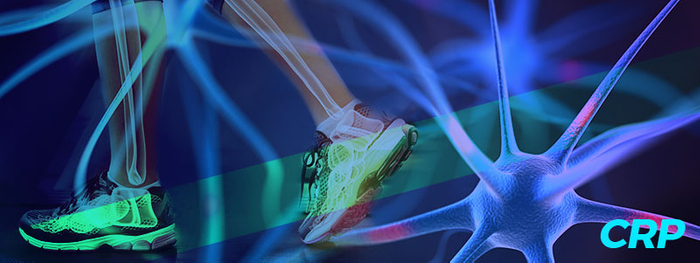
CRP – Inflammation
C-reactive protein, commonly known as CRP, is a ring-shaped pentameric protein in blood plasma. Its levels rise when inflammation has occurred. As athletes often experience higher levels of inflammation due to the impact of physical activity on the body, CRP is particularly important in response to inflammation.
CRP is especially evident in athletes that exhibit more strenuous exercise regimens or those who take part in endurance activity/sports. For non-athlete adults, decreased levels of CRP, TNF-α (tumor necrosis factor-α), and IL-6 (interleukin) occur when taking part in endurance activities.
CRP is released from the liver, adipocytes (fat cells), and immune system cells into the bloodstream within hours of inflammation occurring. Elevated CRP levels have been shown to be prominent in those who’ve suffered intense physical or mental stress. While athletes are well aware of the physical strain they are inflicting on their bodies, our bodies still signal the release of CRP after an intense workout or event, as any sort of physical activity, planned or not, stresses the body.
CRP results from stress and inflammation within the body, making athletes a particularly unique population on which to study CRP’s impact on the body. This is most often brought about by over-exercising, or in endurance athletes that push their bodies to a degree that many athletes (and largely the general population of non-athletes) rarely experience.
Fatouros, Ioannis G. et al constructed in 2006 a study of 17 physically trained men for a 12-week resistance training regimen. This high-intensity regimen consisted of eight resistance exercises that focused on multi-joint impact across four training periods. The team of researchers found that CRP levels increased by 300 percent after the second training period while CRP levels rose 400 percent following the third training period when compared to baseline figures.
While it might sound counterintuitive, researchers found that the best way to reduce CRP levels is to find an exercise regimen and stick to it. The regimens noted involved exercising for 20-80 minutes, 2-7 times per week, including both aerobic and anaerobic exercises.
In short, there was a strong association between reduced inflammation in the study’s participants with the highest rate of CRP level reduction. However, it’s important to note that, just like anything else, too much of a good thing is still too much; moderate exercise that meets your health needs is the best approach to mitigating rising CRP levels.
Sources
https://link.springer.com/article/10.2165%2F11588720-000000000-00000
http://onlinelibrary.wiley.com/doi/10.1113/expphysiol.2014.079335/full
http://physiologyonline.physiology.org/content/25/4/250
https://pdfs.semanticscholar.org/f399/2c83d36f78cc7d056fd418f303ba9020ceb3.pdf
http://clinchem.aaccjnls.org/content/52/9/1820
http://blog.insidetracker.com/crps-reaction-with-your-innerage#
http://commons.nmu.edu/cgi/viewcontent.cgi?article=1011&context=theses
Newsletter
Keep me up to date with the latest news. Find out how you can achieve optimal health through genomics.